Scientists Uncover Magnetism's Long-lost Cousin, Magnetism 2.0!
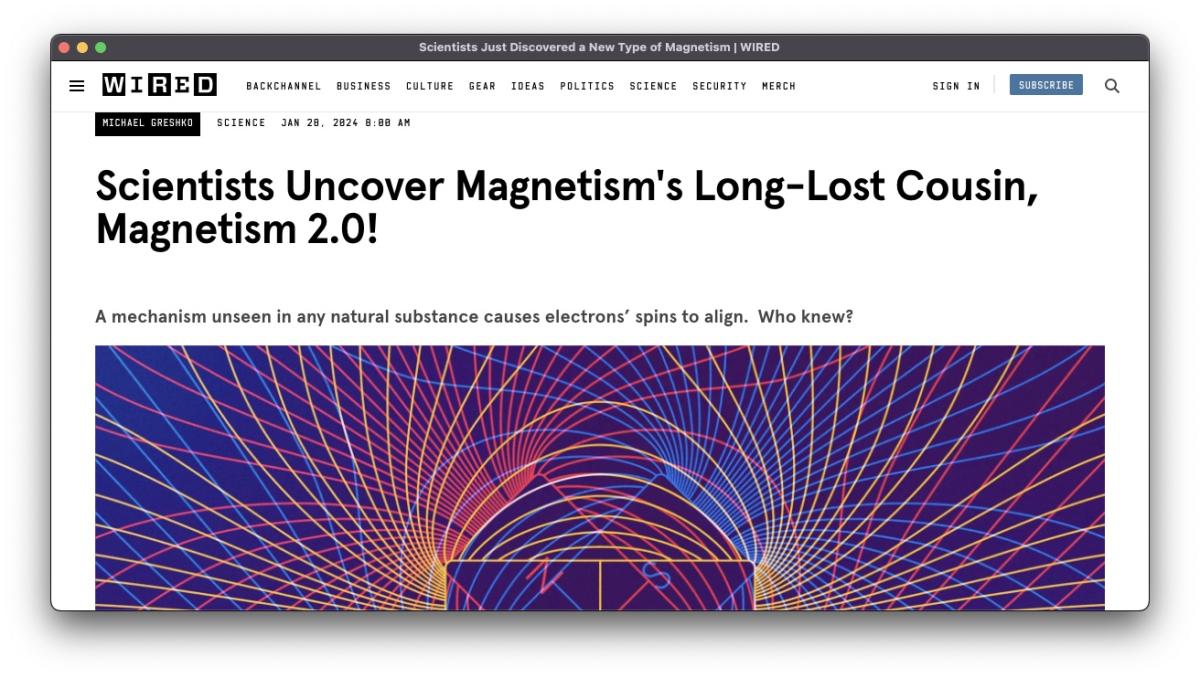
Scientists Just Discovered a New Type of Magnetism
Physicists have uncovered the mystical world of Nagaoka Ferromagnetism, a mind-bending Magnetism where electrons decide to minimize their energy and have a group hug. Forget traditional magnets; this is the avant-garde Electron dance party you didn't know you needed. In a recent episode of "Electrons Gone Wild," scientists engineered a material six atoms thick, because apparently, size matters when it comes to electron shindigs.
Picture this: electrons that can't stand each other, but throw in an external magnetic field, and suddenly, they're all about alignment. The twist? A patterned structure called a moiré lattice – the hottest spot in town for electron drama. Unlike traditional magnet theories, Nagaoka ferromagnetism prefers its material with up to 50% extra electrons, proving that in the world of electrons, more is more.
In the coldest club on Earth (140 millikelvins, to be exact), researchers discovered that these kinetic ferromagnets are the hipsters of the material world. Stay tuned for the next episode, where scientists dream of manipulating particles and achieving the ultimate electron rave – superconductivity. Because who needs practical applications when you can have electron dance-offs?
Physicists have made a breakthrough in the exploration of Nagaoka ferromagnetism, a unique form of magnetism first proposed by Japanese physicist Yosuke Nagaoka in 1966. Unlike traditional magnets, Nagaoka ferromagnetism occurs as electrons within a material minimize their kinetic energy, creating a macroscopic magnetic field. In a recent study published in Nature, scientists observed this phenomenon in an engineered material just six atoms thick, extending the understanding of Nagaoka ferromagnetism.
Traditionally, ferromagnetism arises from the tendency of electrons to repel each other due to their negative charges. In the presence of an external magnetic field, this repulsion can lead to the alignment of electron spins, creating a macroscopic magnetic field within the material. However, Nagaoka proposed an alternative mechanism involving a two-dimensional lattice where each site had only one electron. Removing an electron under certain conditions would cause the remaining electrons to align their spins, resulting in ferromagnetism.
The recent study used moiré lattices, patterned structures formed from 2-nanometer-thin sheets, to create Nagaoka ferromagnetism. These moiré lattices were constructed from monolayers of semiconductors, and the researchers applied weak magnetic fields and varied voltages to observe the alignment of electron spins. Surprisingly, the material exhibited a propensity for ferromagnetism only when it had up to 50% more electrons than lattice sites, contrary to expectations.
The key to this unusual behavior lay in the movement of electrons. As the material acquired more electrons than lattice sites, their delocalization caused the formation of doublons, two-electron combinations. These doublons, seeking the lowest-energy state, created small, localized ferromagnetic regions within the lattice. The researchers discovered that the material exhibited detectable ferromagnetism as the number of doublons increased up to a certain threshold.
While Nagaoka theorized that this effect would also occur with fewer electrons than lattice sites, the experimental results differed due to the geometric characteristics of the triangular lattice used in the study compared to the square lattice in Nagaoka's calculations.
The moiré material was evaluated at extremely low temperatures (140 millikelvins), revealing exciting possibilities for understanding electron behavior in solids. The researchers are now exploring potential applications, including the manipulation of charged particles for superconductivity, based on the kinetic mechanisms observed in the moiré material.